Access Agilent eNewsletter June 2016
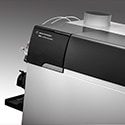
New Agilent 8900 ICP-QQQ delivers increased sensitivity, improved accuracy, and higher productivity
Ed McCurdy and Naoki Sugiyama, Agilent ICP-MS Product Marketing
Tandem mass spectrometry (MS/MS) offers superior performance in many branches of analytical chemistry, with GC/MS/MS and LC/MS/MS being relied upon in organic chemistry labs across many industries. Since the launch of Agilent’s 8800 Triple Quadrupole ICP-MS (ICP-QQQ) in 2012, inorganic (metals) laboratories have also been able to benefit from the performance of tandem MS (ICP-MS/MS).
With several hundred instruments installed in industrial/commercial organizations and research/academic institutes around the world, the Agilent 8800 ICP-QQQ has gained rapid acceptance by the analytical community. The instrument has been used for novel research in publications from the fields of life science and pharmaceutical development, environmental and radioisotope monitoring, food safety, petrochemical analysis, and geochemistry and geochronology [1]. The Agilent 8800 has also been recognized with several prestigious awards from bodies such as ACCSI, Instrument Business Outlook, R&D 100, and SelectScience.
The new, second generation Agilent 8900 ICP-QQQ (Figure 1) provides performance and productivity improvements for real-world applications, with improved matrix tolerance (up to 25% dissolved solids), higher sensitivity, lower backgrounds, more flexible cell gas options, and a new detector with up to 11 orders dynamic range and fast time resolved analysis (TRA) capability.
Leave interferences behind with MS/MS operation
Reaction chemistry provides an effective way to separate the signals of analyte ions and interfering ions, based on their different reaction rates with the chosen cell gas. However, conventional quadrupole ICP-MS has only one mass selection step, in the quadrupole mass analyzer positioned after the collision reaction cell. This means that ions from the sample (and plasma, solvent, etc.) cannot be prevented from entering the cell, so the reaction chemistry is uncontrolled. In practice this means that:
- Matrix or plasma-derived ions (or other analytes) that react with the cell gas will form new product ions. These can overlap the mass used for measurement of an analyte either at its original mass or as an analyte product ion.
- Existing ions that react slowly (or not at all) with the cell gas will remain at their original mass, and may overlap an analyte product ion measured at that mass.
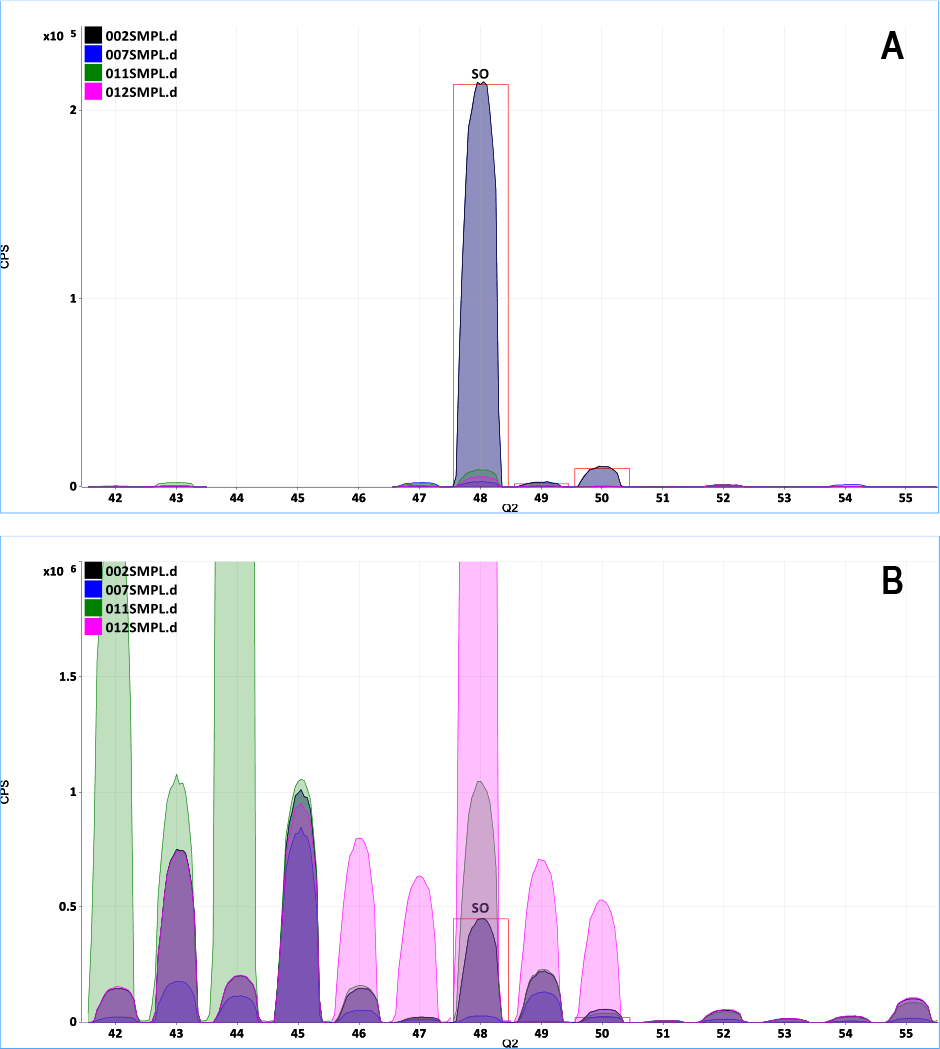
Figure 2. A: Overlaid spectrum of SO+ product ions in MS/MS mode, showing no contribution from co-existing C, Ca and Ti; B: Same overlay in “Single Quad” mode, showing poor isotope template fit and overlaps from ArC+, Ca+ and Ti+ on the SO+ ions.
The Agilent 8900 uses a tandem mass spectrometer configuration with two quadrupoles (Q1 and Q2) separated by the ORS4 collision reaction cell. The first quadrupole (in front of the cell) is used to control the ions that can enter the cell and react. The second quadrupole (after the cell) selectively passes the analyte ions or product ions to the detector. This double mass selection (MS/MS) is the fundamental reason for the superior control of interferences provided by ICP-QQQ.
The performance difference between reaction gas methods using “Single Quad” operation and MS/MS mode is illustrated in Figure 2. The spectra show the effect of two common elements, calcium (Ca) and titanium (Ti), on the analysis of sulfur (S), measured using the typical oxygen mass-shift reaction mode. S+ reacts quickly with O2 cell gas, forming SO+ product ions at M + 16 and thereby avoiding the original polyatomic overlaps on the S isotopes at mass 32, 33, and 34. Figure 2A shows the SO+ isotope pattern measured in MS/MS mode for a 10 ppb S standard, overlaid with a blank matrix of 5% isopropyl alcohol (IPA, blue overlaid spectrum), 1 ppm Ca (green overlaid spectrum) and 1 ppm Ti (pink overlaid spectrum). The IPA, Ca and Ti matrices make minimal contribution to the signal for the measured S peaks, which match the theoretical isotopic abundances (shown by red outline). By contrast, in “Single Quad” mode, the ArC+, Ca+ and Ti+ ions cannot be rejected before the cell, so they remain in the mass spectrum, causing interferences on all the SO+ product ions (Figure 2B).
Exploring Triple Quadrupole ICP-MS for advanced materials and emerging applications
Some of the new capabilities of the Agilent 8900 ICP-QQQ are illustrated in the emerging application of accurate analysis of silica (SiO2) nanoparticles (NPs). Measurement of NPs is important in environmental samples, as well as in assessing food safety and the impact of NPs on human health. NPs are also widely used in industrial processes, manufactured goods and consumer products.
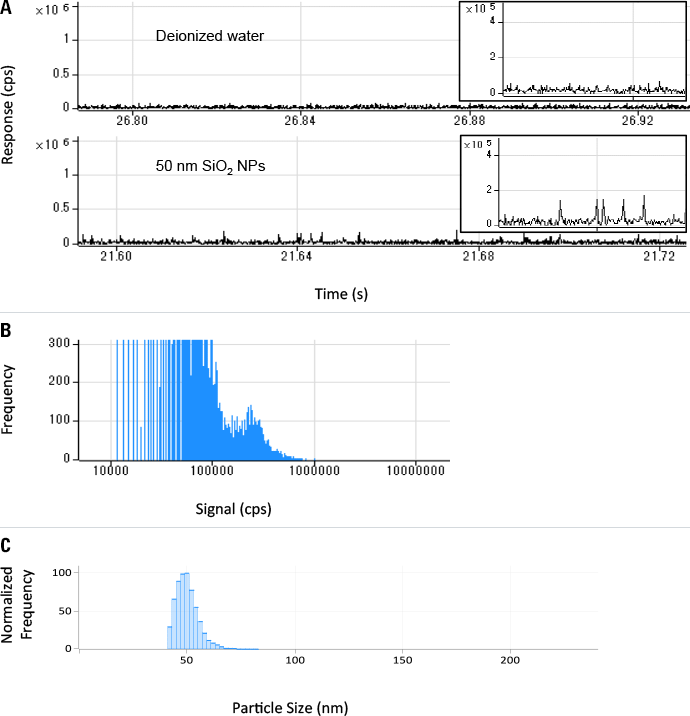
Figure 3. A: Measured signal, B: frequency distribution, and C: particle size for 50 nm SiO2 nanoparticles (40 ng/L).
To characterize NPs, one approach is to measure the signal for each individual NP as it passes through the ICP and is atomized and ionized. This requires a very fast detector response, and the Agilent 8900 ICP-QQQ uses a new detector with fast TRA capability, allowing dwell times of 0.1 ms to be used. The fast detector is combined with specialized software to process the signals and reveal the particle size and size distribution.
Silica NPs present a particular problem for ICP-MS, as the main isotope of Si is measured at mass 28, which can suffer polyatomic interferences from N2 and CO. The Agilent 8900 uses MS/MS mode with H2 cell gas to remove the N2 and CO interferences, allowing accurate measurement of Si at low levels. This ensures that small particles (50 nm or less) can be distinguished from the background signal, allowing unprecedented accuracy for SiO2 NP analysis, as illustrated in Figure 3.
Agilent’s 8900 ICP-QQQ enables you to do more
Agilent’s Triple Quadrupole ICP-MS instruments have opened up new possibilities for analysts. ICP-QQQ allows high purity chemical suppliers and semiconductor manufacturers to certify their products at lower levels. Life science researchers can report absolute quantitative results for proteins and peptides, without the need for compound-specific standards. Food scientists can be confident they are reporting accurate levels of toxic trace elements, even when sample matrices vary. And geochronologists can gain access to isotopic clocks that were previously unusable due to direct isobaric overlaps. All of these applications and more are enabled by the power of ICP-QQQ, using MS/MS to control reaction chemistry.
For more information on ICP-MS technology, please feel free to view this helpful video or contact your Agilent representative today to find out more about Agilent’s ICP-MS systems, accessories, supplies, and software.
Reference
- L. Balcaen, E. Bolea-Fernandez, M. Resano and F. Vanhaecke, Anal. Chim. Acta 894 (2015) 7–19
Agilent ICP-MS Journal
Are you interested in trace metals analysis and eager to keep up-to-date with the latest developments in the field of ICP-MS? Then take a closer look at Agilent’s dedicated ICP-MS Journal. Published four times a year and available as a PDF, you can view the latest issue of the ICP-MS Journal, plus all previous copies, by visiting the ICP-MS Journal Archive.
To receive a personal copy of the ICP-MS Journal direct to your inbox, please sign up here.
Stay informed about the applications that are important to you
Subscribe to Access Agilent
Our free customized
monthly eNewsletter
All articles in this issue
New Agilent 8900 ICP-QQQ delivers increased sensitivity, improved accuracy, and higher productivity
New Agilent 1260 Infinity II LC system delivers higher level of operational efficiency
Robust Agilent 6470 Triple Quadrupole LC/MS delivers confident quantification and streamlined workflows
Achieve accurate analysis of sulfur and nitrogen with the new and simplified Agilent 8355 SCD
Agilent 1290 LC and 6550 iFunnel Q-TOF combine to provide fast, high-resolution peptide mapping of innovator and biosimilar mAbs
Agilent collaborates with Academia—from investigating arsenic mysteries to understanding the complexity of biology
Excellent inertness for analysis of challenging polar compounds
Detection of semivolatile extractables and leachables (E&Ls) found in pharmaceutical products
Agilent multi-omics solutions unravel the effects of low-level gamma radiation on rice seeds
Agilent comprehensive water screening solution reliably identifies wastewater contaminants of emerging concern:
A real-life application
Figure 1
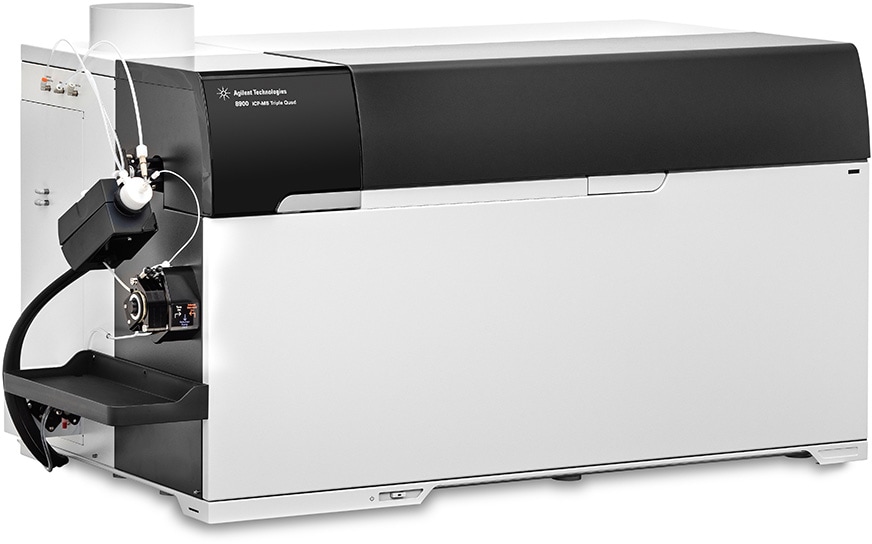
The new, second generation Agilent 8900 ICP-QQQ.
Figure 2
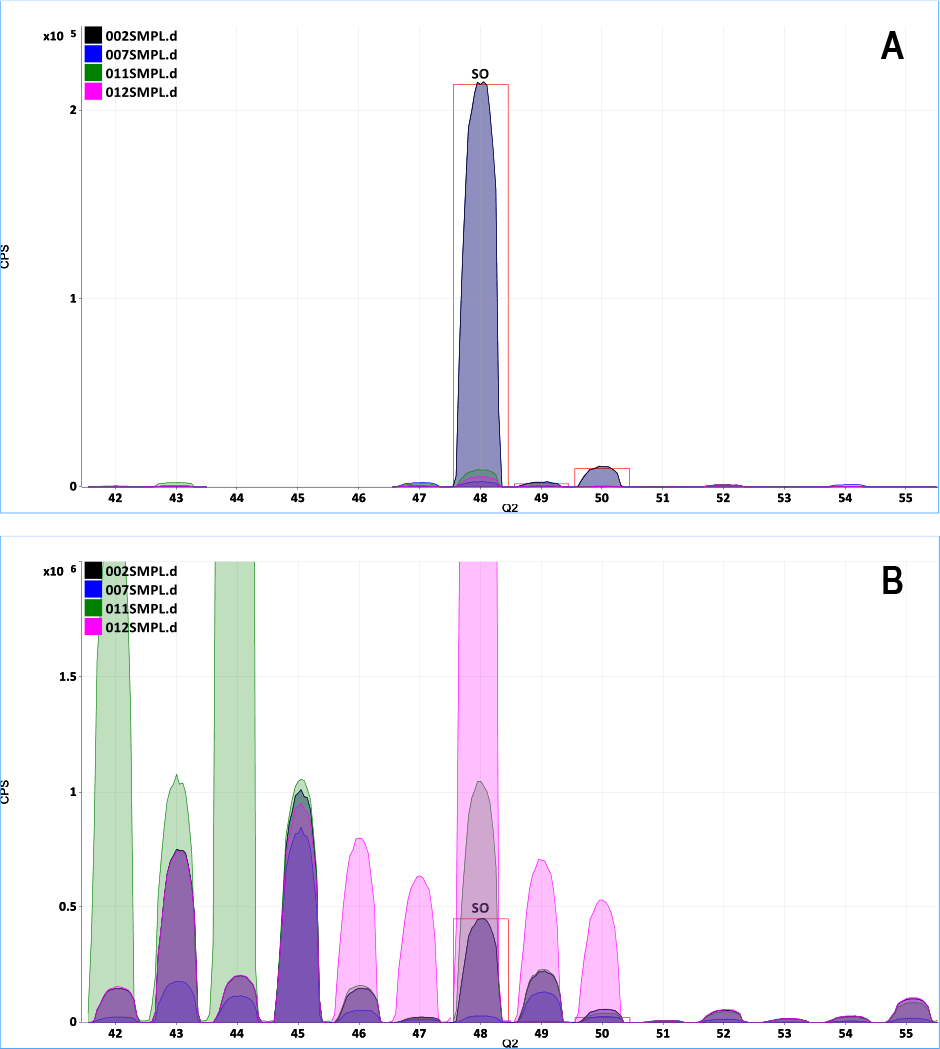
A: Overlaid spectrum of SO+ product ions in MS/MS mode, showing no contribution from co-existing C, Ca and Ti; B: Same overlay in “Single Quad” mode, showing poor isotope template fit and overlaps from ArC+, Ca+ and Ti+ on the SO+ ions.
Figure 3
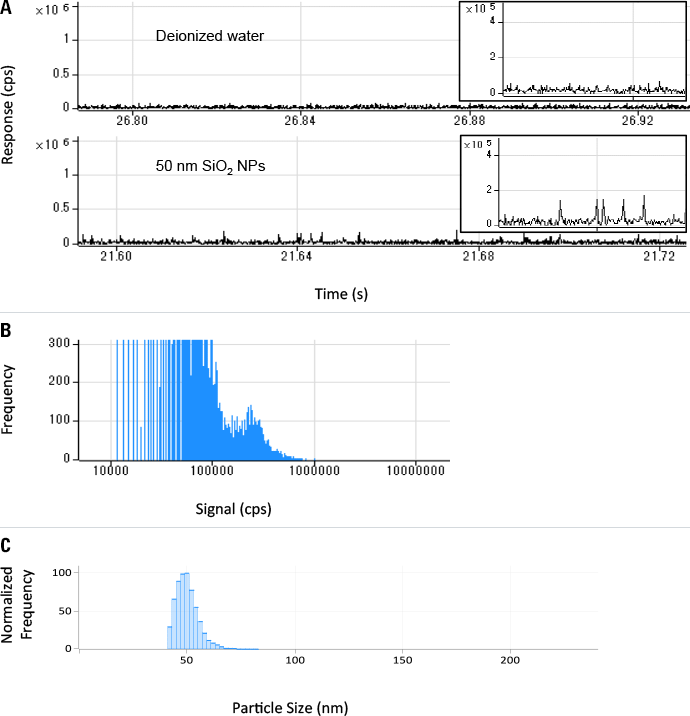
A: Measured signal, B: frequency distribution, and C: particle size for 50 nm SiO2 nanoparticles (40 ng/L).