According to the World Health Organization, approximately one in five people develop cancer in their lifetime.1 Concerningly, mortality rates highlight that approximately one in nine men and one in 12 women die from the disease.1
Since the late 1700s, significant discoveries in cancer research and related fields have been recognized, advancing our understanding of cancer and the exploration of modern methods to help combat the disease.2
Within the last decade, major research milestones have concentrated on cancer genetics, cancer-related drugs and vaccines, and immunotherapy treatments.
In this article, Agilent Translational Research Segment Director, Mark Garner, PhD, sheds light on how cancerous tumors develop in patients, and how research and medicine are advancing to fight them.
Cancer as a mutational disease
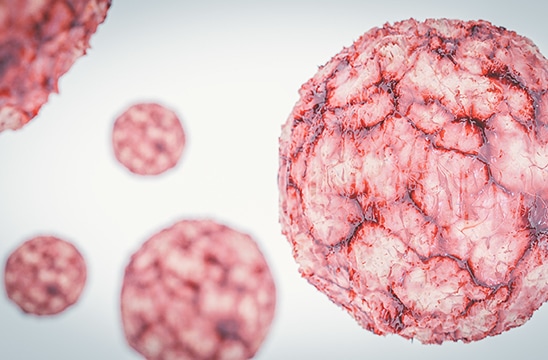
Cancers are caused by mutations in one or more genes that disrupt cellular control mechanisms and lead, directly or indirectly, to uncontrolled cell proliferation, a hallmark in all cancers. Such a mutation is called a “driver” mutation, distinguishing it from the numerous other mutations, known as passenger mutations, which cancer cells accumulate.
In the 1970s, the mutational basis of cancer was established with the identification of genes that, when mutated, drive cancer development. These genes are referred to as “oncogenes”, the first of which was identified in the Rous Sarcoma Virus, a virus that causes cancer in birds.3 Having identified the viral gene (src) responsible for causing the cancers in birds, Varmus and Bishop showed that a src gene analog was naturally present in all bird species they examined. This finding demonstrated that oncogenes are a normal, essential part of the cellular genome, and mutations in them disrupt finely balanced cellular homeostatic mechanisms.3
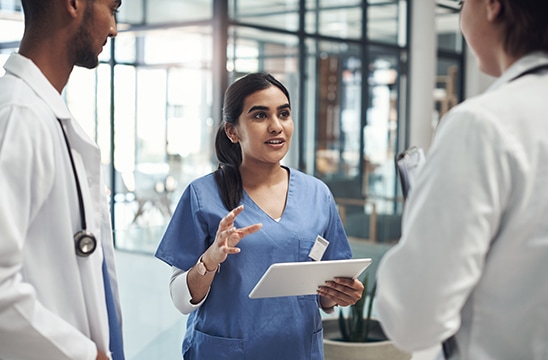
Identifying mutations or other genomic disruptions present in the tumor is an essential part of cancer diagnosis. Molecular Tumor Boards (MTBs), comprising cancer genomics experts and other specialists, integrate genomic and molecular data on the patient’s tumor with clinical observations to recommend possible treatment options to the patient’s oncologist.
MTBs are now considered a standard of care in many cancer centers and represent the clearest realization of the concept of “precision oncology”—targeting a patient’s tumor based on its molecular phenotype rather than its organ of origin.
Precision oncology has fundamentally changed the way researchers and clinicians approach cancer. The concept and promise of precision oncology lies in targeted therapies, especially those based on a detailed molecular characterization of the patient’s tumor, are expected to be more effective and have fewer adverse effects than conventional chemotherapies.
Other than gene therapies, cancer treatments do not target a particular gene per se, but rather the protein product of a gene, another protein in a signaling pathway, or even a small molecular metabolite susceptible to abundant changes because of the tumor mutation. Identifying an effective molecular target for cancer treatments is often a key challenge faced by researchers and clinicians.
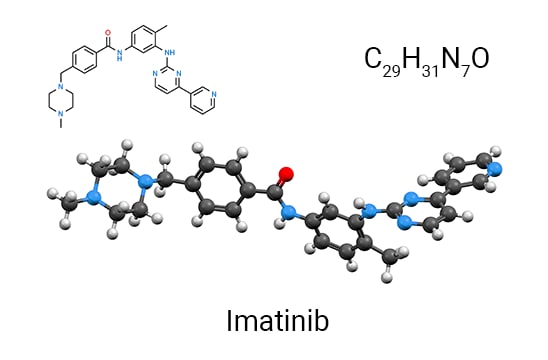
A classic example of this gene-protein-drug paradigm is the development of the drug “Gleevec” to target Chronic Myelogenous Leukemia (CML). Before Gleevec, only 30% of patients with CML survived up to five years after receiving the diagnosis.4
In the early 1960s, it was noted that most CML patients had an unusually short chromosome 22, known as the “Philadelphia” chromosome. Initially, it was assumed that the end of the chromosome was deleted. However, in 1973, Janet Rowley, through meticulous microscopic examination, revealed that the missing segment of chromosome 22 was in fact a translocation, and the “missing” piece was appended to the end of chromosome 9.5
Once molecular techniques became available and widespread, progress accelerated. The DNA recombination predicted by the chromosomal translocation was validated. It was observed that during the process of recombination/translocation, by random chance, the abl gene from chromosome 9 is fused to a segment of the bcr gene at the end of chromosome 22. This fusion forms a new gene: bcr-abl (as highlighted in Figure 1).
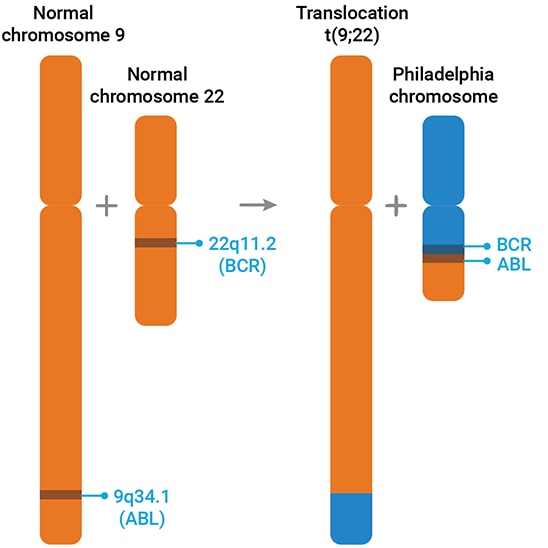
The abl gene is similar to the cellular analog of the src gene and codes for a tyrosine kinase protein. Like most kinases, it is part of an intricate network of signaling pathways that regulate various cellular processes, including division. Fusing part of bcr to abl disrupts this autoregulation, and the hyperactivity of the bcr-abl tyrosine kinase then disrupts cellular control, leading to CML.
Research led by Dr. Brian Drucker and Dr. Nicholas Lyndon focused on screening compounds that would inhibit bcr-abl without affecting normal cellular function. One promising compound, STI-571 (Gleevec), advanced to a phase I clinical trial. In a New York Times interview, Dr. Druker commented, “During clinical trials, we saw this miracle: once the patients were up to effective doses, we got a 100 percent response rate.”6 Eventually, Gleevec gained FDA approval and has since saved, and continues to save, many thousands of lives every year, including those affected by nine other forms of cancer driven by the same kinase.
However, the discovery of an effective cancer drug treatment is not always a linear journey. For instance, the plenary session at the AACR 2023 meeting was titled, “Beating KRAS: A 30-Year Overnight Sensation” in recognition of the long, difficult path from identifying KRAS as a human oncogene to being able to target the K-RAS protein in a clinically useful way.7
The immune system and cancer: predator and prey, chance, and necessity
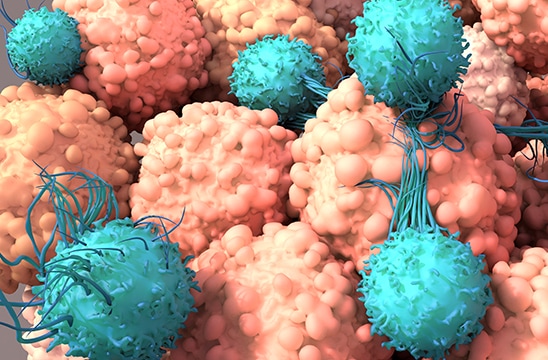
Cancer immunotherapy is designed to enhance the ability of a patient’s own immune system to act as a “predator” for cancer cells. It is based on the concept of immune surveillance, which is a fundamental function of the mammalian immune system, whereby natural killer (NK) and macrophage cells of the innate immune system work in synergy with adaptive T cells. Together, they actively target and destroy cancerous cells preferably at an early stage prior to tumor formation.
However, the target of cancer immunotherapy is an elusive prey, capable of adapting its environment to hide and even disable the immune system. Of course, neither cancer cells nor immune cells “think” or have intention. In reality, a cancer cell carries all of the genetic mutations it has undergone and continues to divide rapidly, accumulating more random mutations as it thrives. These mutations drive its cellular metabolism and interactions, actively shaping its environment to evade the immune system.
Jacques Monod, one of the founders of molecular biology, considered this dichotomy of a random mutational processes and the theory of undergoing what appeared to be an intentional change to evade the immune system. In the philosophical treatise Chance and Necessity, he explained that the mediator between the two is “natural selection,” which eliminates most organisms that have undergone silent or innocuous mutations.8
For example, if you plate ampicillin-sensitive E. coli on typical LB agar plates with ampicillin added, nothing grows. However, if you grow the same liquid culture through a few cycles and repeat the plating, eventually, some ampicillin-resistant cells start appearing. These resistant cells were not “prepared.” It is through random mutational processes that the ampicillin-sensitive E. coli acquired the necessary mutations to become resistant to the antibiotic on the plate—chance and necessity. The mediator is natural selection, which “selects” those lucky few cells. It may resemble a conscious, deliberate choice by an organism to acquire a characteristic, but it is based on random selection.
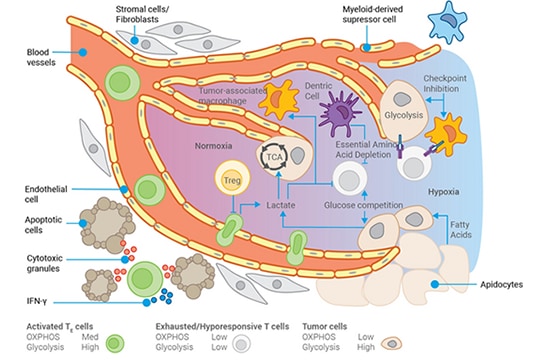
In tumors, similar processes are occurring. One consequence of the accumulation of mutations by cancer cells is the derangement of cellular metabolism, which is necessary to create precursors for macromolecules to sustain the rapid proliferation of tumor cells. Tumor cells can use almost any carbon source to fuel metabolism, even degrading RNA to access fuel. These changes impact the extracellular environment around the tumor, known as the tumor microenvironment (TME).
Tumor cells may consume almost all the glucose from the TME, forcing infiltrating T cells to rely on lipids as fuel. Depending on the type of lipids, this can attenuate their antitumor activity. The TME often becomes more acidic and lower in oxygen due to insufficient blood vessels to support the tumor’s rapid growth.
The TME is comparable to a toxic waste dump, which weakens the immune system’s ability to locate and destroy tumor cells. It's almost as if the tumor cells instinctively protect themselves by altering the TME.
Furthermore, like normal cells, the tumor recruits fibroblasts to excrete collagen and form an abnormal extracellular matrix, but the cancer cells also create a matrix. Paradoxically, even though the tumor microenvironment is full of immune cells, these immune cells have decreased or eliminated their ability to act as effective predators. As a result, their prey, hiding in plain sight, lives for another day.
Shots on target
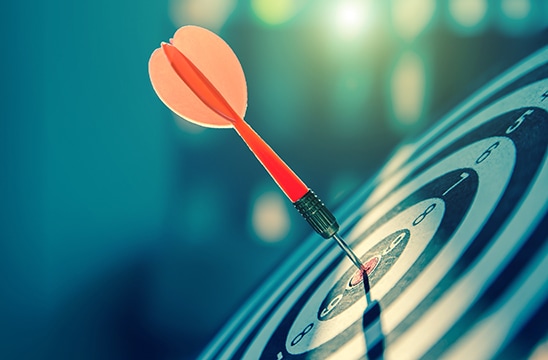
T cells recognize cells as cancerous by interrogating peptide fragments (antigens) presented on the cell surface. In development, T cells learn to recognize antigens from all of a person’s “own” proteins. When a T cell encounters a cell with a previously unknown antigen (a new or “neoantigen”), the T cell recognizes it as foreign, cancerous, or infected (this same mechanism is how virally infected cells are identified) and initiates cell killing.
Recently, it has been acknowledged that tumors with a high mutational burden must present more neoantigens to the immune system. Sometimes, patients with tumors that have a higher mutational burden respond better to immunotherapy than those who have tumors with fewer mutations. The interpretation is that the greater the number of mutations, the greater the number of neoantigens, and the higher the chance that a random T cell will recognize one of the neoantigens and target the tumor cell. The inevitable metaphor is that the immune system has “more shots on target” and is more likely to eliminate the cancer.
In 2020, the US FDA approved the anti-PD-1 drug pembrolizumab (Keytruda) for use against any solid tumor with a mutational burden higher than 10 mutations per megabase. Subsequent studies have questioned whether a single cutoff for all tumor types is optimal, but the principle that highly mutated tumors are more susceptible to the immune system is clear. Provocative suggestions have even been made to induce more mutations in tumors, to make them more susceptible to the immune system. While many still consider this a radical approach, we are learning how to effectively and safely use the mutations that create cancers to our advantage in order to destroy them. As Sun Tzu said in The Art of War, “To secure ourselves against defeat lies in our own hands, but the opportunity of defeating the enemy is provided by the enemy himself.”10
About Mark Garner, PhD.
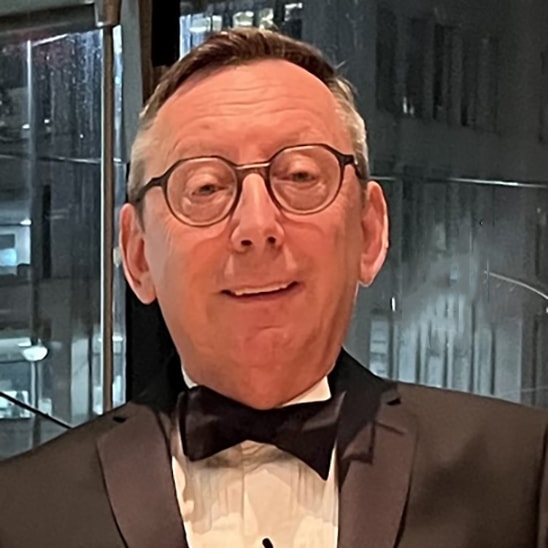
Mark Garner received his PhD in Biochemistry from Michigan State University. As part of his graduate work, he invented the now universally used gel-shift or “EMSA” assay for the study of protein-DNA interactions. He was then successively an American Cancer Society Postdoctoral Fellow and National Research Service Awardee at the NIH, as well as doing a second postdoc at the University of Wisconsin-Madison. During this time, Mark also developed a long-term relationship with the Max Planck Institut für Biophysikalische Chemie in Göttingen. He then returned to the NIH where he carried out fundamental research on capillary electrophoresis of nucleic acids and the molecular biophysics of macromolecular crowding and its effects on cellular processes. Since entering the private sector, he has been a key member of several cutting-edge companies. In 2006, Mark relocated to Toronto, Canada where he currently lives. He joined Agilent Technologies in early 2020 and is currently director of Agilent’s Translational Research Marketing Segment.
References
1 Global cancer burden growing, amidst mounting need for services (who.int) 2 Milestones in Cancer Research and Discovery - NCI 3 Varmus, H.E. Viruses, genes, and cancer. I. The discovery of cellular oncogenes and their role in neoplasia. Cancer 55, 2234-2238 (1985) 4 Pray, L. (2008) Gleevec: the Breakthrough in Cancer Treatment. Nature Education 1(1):37 5 ROWLEY, J. A New Consistent Chromosomal Abnormality in Chronic Myelogenous Leukaemia identified by Quinacrine Fluorescence and Giemsa Staining. Nature 243, 290–293 (1973). 6 Researcher Behind the Drug Gleevec - The New York Times (nytimes.com) 7 AACR Annual Meeting 2023: Beating KRAS - American Association for Cancer Research (AACR) 8 Monod, Jacques. Chance and necessity : an essay on the natural philosophy of modern biology. London, Penguin, 1997. 9 Marcus et al FDA Approval Summary: Pembrolizumab for the treatment of tumor mutational burden-high solid tumors Clin Cancer Res 27, 4685-4689 (2021) 10 Tzu, Sun. The Art of War. United States, Shambhala, 2019.